The water cycle for crops, grass and forests includes rainfall and other types of precipitation, then water landing on plants and soil, entering soil, being evaporated from wet surfaces, taken up by plants and moved back to the atmosphere; and then leaving the land as surface flow or drainage to join groundwater, streams, rivers and lakes.
The complete water cycle for a country or region includes many other things – reservoirs, public water supply, urban drainage, sewage and sewerage, industrial usage, recycling from ‘green’ to ‘grey’ water, effluent discharge into rivers and seas. Efficient and fair distribution of water relies on diligent management, which is now governed by an Act of Parliament [1]. This article concentrates on those parts of cycle that affect sustainable food production.
[draft subject to editing]
[CC7 in Climate and Crops – back to Climate and Crops summary page]
Summary
- The region is highly variable in land use – upland tracts, forests, livestock, arable farming, horticulture, industry, human habitation – yet individual units are interconnected. Successful water management therefore needs to attend equally to units individually and to large groupings such as catchment and river basins.
- Priorities for land management in agriculture and forestry are to ensure adequate soil water stores that allow growth during periods of low rainfall, to maintain controlled drainage to reduce the time soil is flooded or saturated, and to limit uncontrolled surface runoff and drainage to neighbouring land, human habitation and waterways
- The means to regulate water flows and stores have been known for centuries. Scientific modelling can predict fairly accurately the partition of flows, for example between evaporation and drainage. Safeguarding the future will rely less on innovation than on restoring and correctly applying well established methods.
A varied country
Very little land in Scotland is in its ‘natural’ state after the last retreat of ice. Most has been transformed by human intervention over the past 5000 years. While land might change in the future – through for example re-wilding and afforestation – what we have now is what was shaped by previous occupants.
For any type of land, the various water flows and stores are highly sensitive to land management. The consequences for sustainable food production of any future change in rainfall will depend on how management today can restore and then regulate flows and stores. To achieve this will need action at each individual unit of land and then across all units collectively.

Fig. 1 Map showing agricultural fields and larger holdings, some of the smaller coloured blue for visual effect, the right hand blocks showing (upper) a lowland area covered by many small fields and (lower) a transition from upland to lowland. Data from GS Begg and colleagues, James Hutton Institute [2].
Units of land differ greatly in size across the country (examples Fig. 1). Most of the productive agricultural land is visible on the map as darker areas to the east, centre and south of higher land (light brown). Each field or unit of pasture is given a boundary line, and as fields become smaller, the multiple boundaries create a shading effect.
The square image upper right is extracted from an area of high field density: some fields are coloured to emphasise the small size of individual units. (The average field size in lowland Scotland is about 7 hectares.) The image lower right shows transition from an upland area, consisting of a few very large units, to a lowland one of many small units. As upland transitions to lowland, river valleys become marked out by the smaller fields formed on the flatter land by the waterways.
The shape or topography of the land forces waterways to form river catchments. The parts of catchment are connected – what happens higher up affects things lower down, while different forms of lowland land use might compete for the water they receive. In some places today (and ideally, in the future, everywhere) the water cycle is managed at the scale of the catchment [3].
Defining the cycle – inflows, storage and outflows
The stores and flows of water can be expressed in the same way for any type of land use (Fig. 2). Precipitation falls on vegetation and soil, which intercepts and then stores a portion of the water. Excess either runs off as surface flow or moves down as drainage through the soil to depths out of reach of roots. Flows of both types may eventually combine into streams and rivers or remain in rock strata as ‘groundwater’.

Fig. 2 Rain (or snow or mist) falls on land. A part of the water is held by vegetation and soil, while the rest flows out as evaporation, runoff along the land surface and drainage through the soil. Diagram: www.livingfield.co.uk, image – Sidlaw Hills to Carse of Gowrie [4].
When measuring or modelling the water cycle, stores and flows can be seen as entities defined by an amount of water they can hold and the rate of movement into and out of the entity (Fig. 3). The water intercepted by vegetation either evaporates to the air or flows or drips from leaves, stems and branches to the soil below. Some of the water reaching the soil, whether directly or from vegetation, evaporates directly back to the air, some enters the soil and some may flow away along the surface.
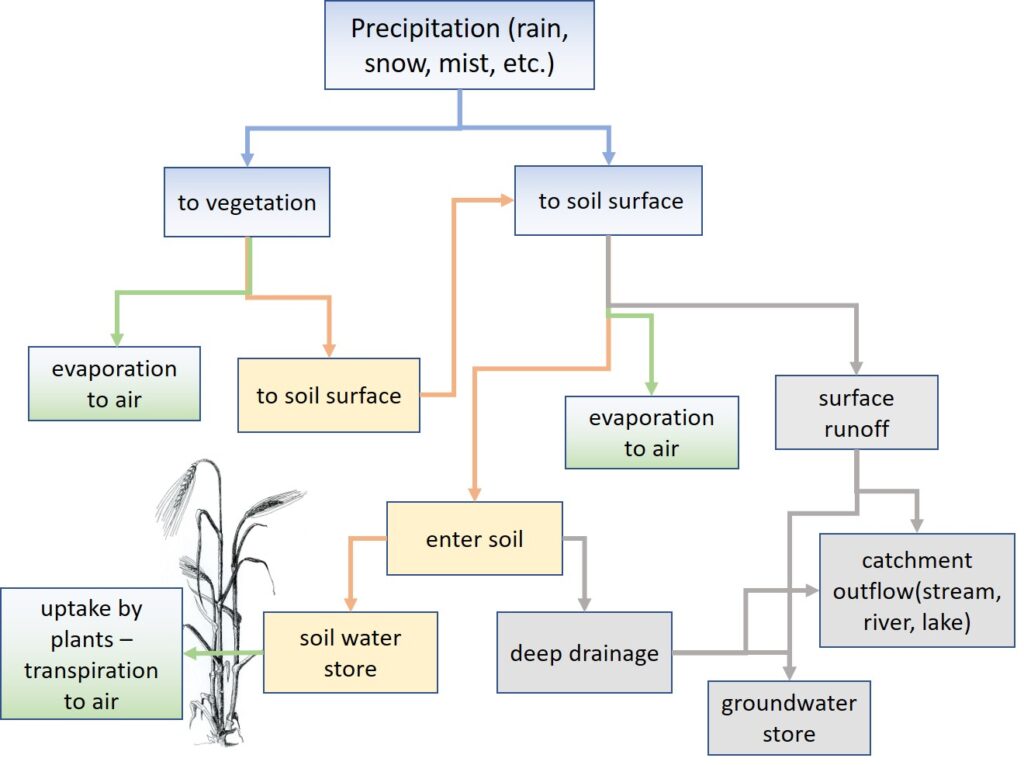
Fig. 3 Diagram of water stores and flow pathways in the water cycle of productive land (www.livingfield.co.uk).
Water entering soil moves through various channels and fills pores where it can be retained under gravity. This is the soil water store – essential for most plant life on land. The size of the store is a crucial factor in determining the productivity of land and in particular its capacity to sustain plant growth during drought. It is determined by the depth of soil and its capacity to retain water (volume water per volume soil).
The key to successful soil water management is to achieve a balance between the soil water store, surface flow (runoff) and deep drainage. When water is in short supply, then the aim is to ensure as much as possible enters the store, and little is lost as runoff and drainage. The problem often faced in dry climates is that the soil either become compacted, increasing surface flow after rain, or forms cracks and channels through which rainwater passes without entering the soil store.
At the opposite extreme, where it is too wet, the store fills, then overfills , forcing out the air that plant roots need to function. Traditionally, sub-surface drainage systems (e.g. fired clay pipes) were introduced, many in the 1700s and 1800s, to ensure excess water was allowed to drain away, but many such systems have not been maintained. Sometimes, there is so much rain and surface flow from higher land that fields become waterlogged whatever drainage is in place.
An example
The generalised stores and flows in Fig. 3 vary in size with climate, geology, soil, slope and vegetation. In many regions, the stores and flows do not all operate continuously. An example in Fig. 4 is for land holding a vegetation of small trees, shrubs and unmanaged grazing, interspersed with areas of bare soil. Most roots penetrate the upper, red layer of soil: the soil store is here defined by this root zone.
Some rainfall is intercepted by the vegetation (V) while the rest lands directly on the soil surface (S). Of that intercepted by vegetation, a part evaporates directly to the air (Ei) while the rest runs down to the soil. That reaching the soil can move three ways – into the soil, to the air by evaporation (Es) and – if the rain is heavy and soil compacted – flow across the soil as surface runoff. Water held in the root zone can be taken up and transpired by plants to the air (Et).
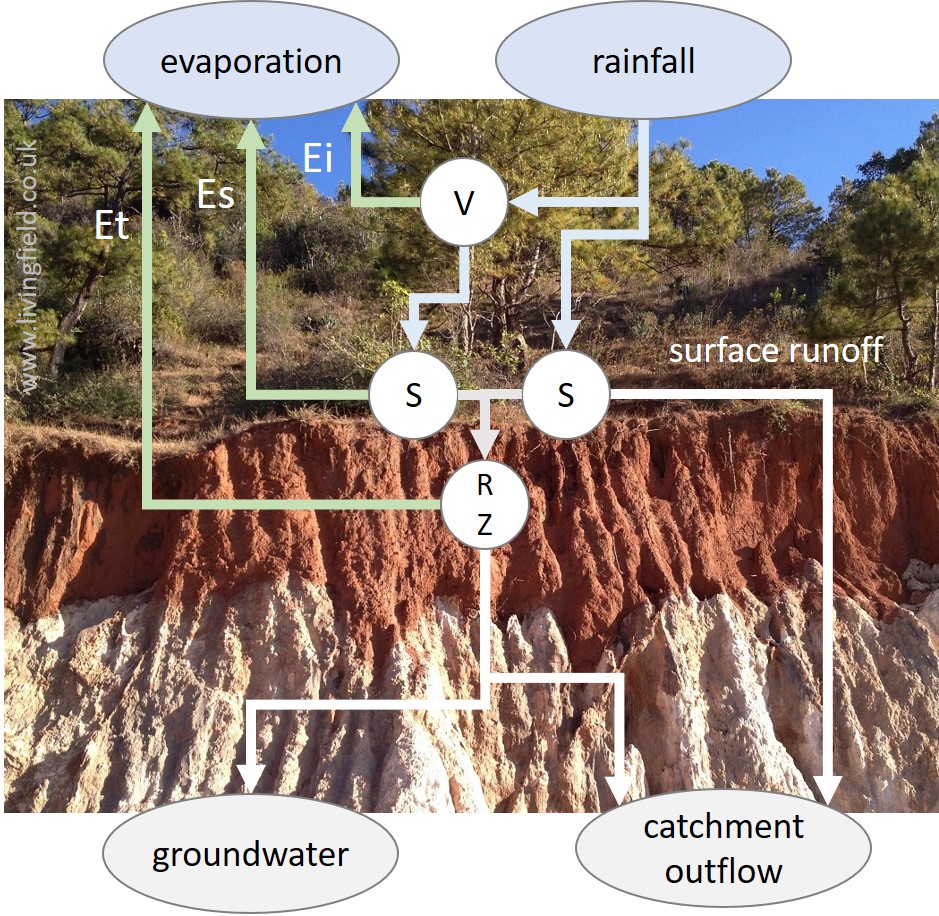
Fig. 4 Water flows shown on a vertical section through vegetation, soil and rock. Rainfall is intercepted by vegetation (V) and falls on the soil surface (S), where some enters the soil store, delimited by the root zone (RZ), and some leaves as runoff and deep drainage. Text describes evaporation of water by Es, Ei and Et. Original photograph of landscape in Burma/Myanmar by the author (www.curvedflatlands.co.uk).
After a period of rainfall, all the flows in Fig. 3 and Fig. 4 would likely be active. It had been dry for some time before the photograph was taken and most flows had decreased to zero. The only ones operating, and even then slowly, were uptake from the root zone and transpiration (Et) through foliage.
Use of water by plants
To function – to grow and yield – crops, grass and trees need to be hydrated or ‘full’ of water. (A potato for example is about 80% water.) To achieve hydration, their roots take up water from the soil. However, to grow, plants need to take in carbon dioxide or CO2 from the air and convert it to plant matter using solar energy (the process of photosynthesis). Their cuticle or outer skin is too impenetrable for CO2 to pass through, so plants open the stomatal pores in their leaves to allow CO2 gas to enter by diffusion. When the pores are open they also lose water to the air, and so to remain hydrated, plants have to take up more water from the soil store. This movement from soil to air is known as the transpiration stream (Fig. 5).
The constant need for soil water by growing land plants puts a major limitation on where crops, grass and trees can grow and how much they grow. Typically, more water passes through the plant in transpiration than CO2 is incorporated in photosynthesis: the ratio varies with the humidity of the air, leaf temperature and the type of plant but is typically of the order of 1000 – for each kg of dry matter produced by a plant, 1000 kg of water is transpired. This transpiration also has a role in cooling leaves exposed to intense solar radiation.
Of the three sources of evaporation in Fig. 3 and Fig. 4, plants can increase their weight only through transpiration (Et). Evaporation from the soil surface (Es) and from that intercepted by vegetation (Ei) should not all be viewed as wasted since it helps to regulate the temperature of the whole soil-plant system.

Fig. 5 Diagram of water flows for a single plant. Growth by intake of CO2 from the air occurs only when water is taken up by roots and transpired through leaves. Water intercepted by plant surfaces may aid cooling but does not contribute to growth directly.
Conclusions for the north east Atlantic region
The capacity for high yield in regions with a Long Cool Summer [5], like lowland Scotland, relies on there being (a) enough rainfall to ensure soil stores are filled or replenished and transpiration unimpeded throughout the summer period of high solar income, and (b) not too much rainfall so that soils are waterlogged.
The range of possible interactions between climatic change and the water cycle means that – as for temperature – it is not possible to give simple and certain predictions as to the effect of a specific change in rainfall on the productivity of land. Some lessons can be learnt from years that receive too little or too much rain (the subject of future articles), but the long term effects of systematic change in rainfall amount or pattern need careful interpretation.
Whatever the future trends in rainfall, land policy and management will be better able to cope if they encourage and implement practices that regulate the water cycle at a range of scales. They include –
- Root zone management – cultivation of soil to maximise water holding capacity (commonly related to organic matter content) and to ensure that roots of crops and grasses can penetrate and explore the full depth of soil [6].
- Surface runoff – good root zone management to aid penetration of rainfall coupled with flow-reducing techniques such as minimum tillage and retention of crop stubble, tied ridging, vegetated wheel tracks and cultivation across the slope.
- Vegetation cover – maintenance of plant cover for most of the year, for example by perennial cropping and grazing, relay cropping and various forms of mixed cropping.
- Field to field flows – including maintenance of field drainage systems to remove excess water from the root zone and various forms of bunding and vegetated field margins to prevent or reduce surface flow out of a field.
- Catchment integration [3] – to promote fair and efficient management of stores and flows among different types of land use, for example, to reduce flooding or water shortage downstream.
The next article will examine records of rainfall in the region and point to those patterns and quantities of rain that damage or limit production. After heavy rain, fields like those in Fig. 6 are not uncommon – though for future security, they should be.

Fig. 6 Fields in Perthshire, waterlogged and pooled after heavy rain, wheel tracks visible (www.livingfield.co.uk).
Sources | References | Links
[1] Scottish Government: Implementation of the flood risk management (Scotland) Act 2019 – includes Integrated Catchment Management.
[2] Mapping of the type in Fig. 1 is produced by various groups at the James Hutton Institute, Dundee and Aberdeen. The one shown was derived from IACS – the EU Integrated Administration and Control System – by the Agroecology group at Hutton Dundee, work of GS Begg, N Quesada, GR Squire; enquiries to graham.begg@hutton.ac.uk.
[3] Integrated catchment management. Example: Tweed Forum – catchment management. Value for wildlife and habitat: Scottish Wildlife Trust’s Integrated Catchment Management (policy document), available as a PDF file. Analysis: James Hutton Institute report – Waylen K, Marshall K, Blackstock K. 2019 Reviewing current understanding of catchment partnerships.
[4] The small sub-catchment shown in the photograph is one of many that flow into the Carse of Gowrie, an area of marsh, drained many centuries ago to give land of high agricultural productivity. The photograph was taken from an area of higher land consisting of large agricultural holdings, supporting wooded gullys, rough grazing, managed grass and some arable (see Fig. 1), looking approximately south-east towards the Tay estuary. The lower land supports the high-input arable (cereal, potato, vegetable) and grass of farms near the estuary. Such highly variable landscapes are typical of eastern Scotland. For optimum management, the water cycle needs attention in every field and at every field boundary.
[5] A previous article explains the Long Cool Summer effect which leads to consistently high yields in this region.
[6] One of the positive – but unsung – effects of intensification between 1950 and 1980 was to increase the routine ploughing depth from 10-15 cm to 30-40 cm and hence break up sub-surface hard pans and increase the volume of soil readily available to roots. On the other hand, too- heavy ploughing and soil mincing (common in arable systems today) destroys soil microbial functioning and causes of loss of organic carbon, which in turn reduces water holding capacity.